ATOMIC STRUCTURE, PERIODIC TABLE, ELECTRONIC CONFIGURATION, ELECTRON TRANSITIONS: ELECTROMAGNETIC SPECTRUM AND CHEMICAL BONDING
Periodic table| Atomic structure and electronic configuration
Periodic table is formed when atoms of elements are arranged in a tabular form. This is based on the number of protons in their nucleus. Moreover, this is also directly related to the number of electrons in the outer shell of the neutral atom. It also determines the chemical behavior of elements.
The nucleus of the atom also contains neutrons and protons, and they determine most of the weight of the atom. Protons have positive charges but electrons have negative charges and then neutrons have no charge (neutral).
Simple basic classical diagram of the atom
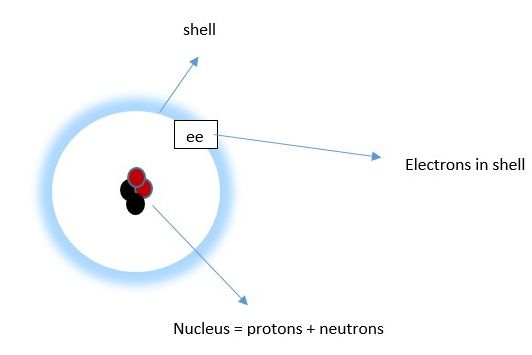
Picture of periodic table
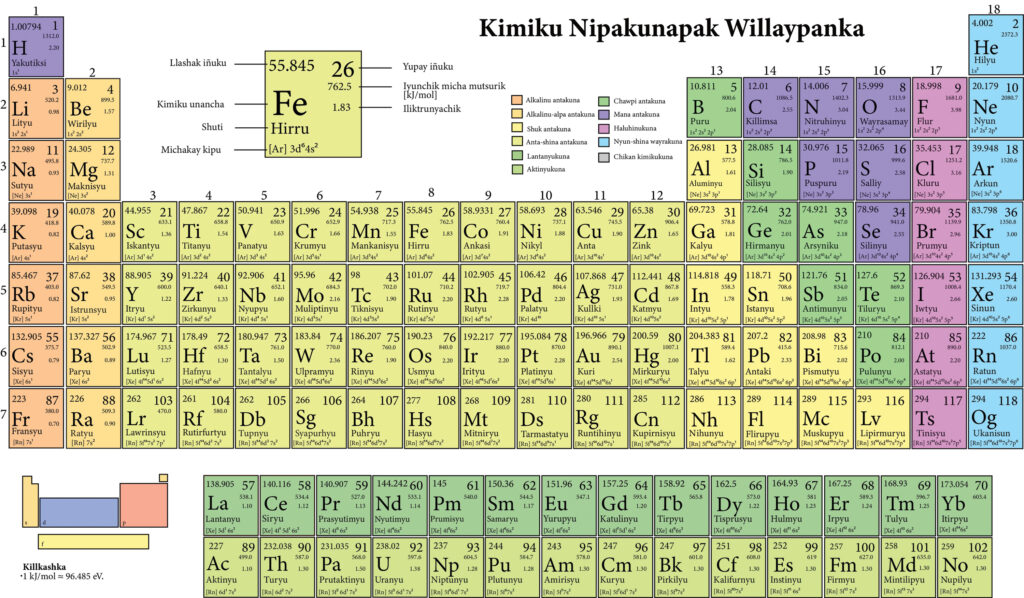
Simple representation of Lithium atom showing protons and neutrons at the center surrounded by electrons(grey).
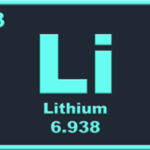
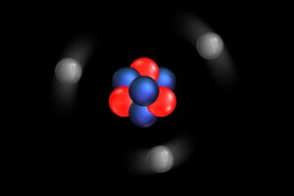
Electron configuration:
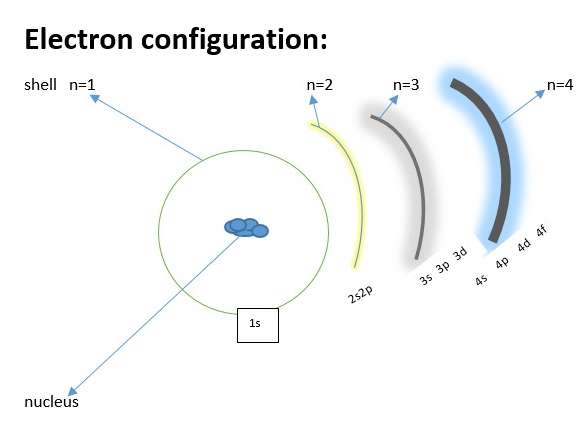
Electrons found in orbitals [s, p, d, f, g) An orbital is a space where there a probability of locating an electron in the shell of an atom. The number of electrons in a shell is determined by the formula 2n2 (where n is the shell number). The shell or the shell number n is the principal energy level of the electron in the atom. The first shell next to the atomic nucleus is shell number 1 (n=1). This shell can contain a maximum of 2 electrons. [ 2 x 12) = 2. The next shell n= 2 can contain a maximum of (2 x 22 )= 8 electrons. The next will be a maximum of 18 for n = 3 shell, 32 for n = 4 shell etc.
There are different SHAPES of electron compartments of sub-shells or sub-levels or orbitals within a shell, and these are caused by the angular momentum quantum number (l) of the electrons in the atom. There is s-subshell level orbital, p-subshell level orbitals, d-subshell level orbitals, f-subshell level orbitals, g etc .and these are identified by the angular momentum quantum numbers in the formula [l = 0… to n-1]. The first shell n=1, l=(1-1)=0 implies l can have only one value of zero in the first shell n=1 and that is the s subshell level orbital which is spherical in shape and there is only one s-orbital in every shell. However, the second shell n= 2, l= 0 and l=(2-1)=1, implies l has two values and we have two subshell levels (s, l=0 and p, l=1). The p orbitals are shaped like dumbbells. The third shell n=3 , has l = 0 (s) and l=(2-1)=1(p), up to l= (3-1)=2 (d) implies three values of l which are 0,1,2 which correspond to subshell level orbitals s, p and d present in shell number 3. The d orbitals are shaped like donuts. The fourth shell n= 4 , l= 0,1,2,3 implies four subshell levels (s (l=0), p (l=1), d(l=2), f (l=3)) present in shell 4 of the atom. However, these subshell levels become bigger and more diffused and overlap as the atomic size increases for n values above n=3.
The NUMBER of orbitals in a subshells level is obtained from the magnetic quantum number of the electron (ml). This is given by the formula ml =-l to +l. This means at the s subshell or level, l =0, ml +-0 that is only one value for ml in this case implies there is only one orbital in an s-subshell or level or simply say there is only one s orbital. However, at the p subshell level l =1, ml = -1,0+1=3 values, there are 3 orbitals in a p-subshell level or simply say that, there are three p-orbitals or subshells[px,py,pz]. If we look at the d subshell level, l =2, ml= -2,-1,0+1,+2 values, implies there are 5 orbitals in a d subshell level or simply say that there is five d orbitals or subshells [dxy, dyz, dxz, d x2–y2 and dz2]. Finally at the f subshell level, l=3 , ml= -3,-2,-1,0+1,+2+3 values implies there are 7 orbitals in an f subshell or level or simply say that there are seven f orbitals or subshells.
Pauli’s exclusion principle : Each orbital in the atom can contain a maximum of two electrons. Two electrons in an orbital should have opposite spins. Therefore, the spin quantum number (ms) of the electron in an orbital is given a value of +1 . e.g., box represents an s orbital containing two electrons with opposite spin directions.

Putting all together let’s write the electron configuration for some elements.
Hydrogen = 1H number of electron = 1 . Electron configuration = 1s1 . The number 1 in front of s represents shell number 1. The s is the orbital since shell 1 has only s orbital. The superscript 1 on s represents the number of electrons in the s orbital which is one in this case.
Helium = 2He = 2 electrons. Therefore, electron configuration is 1s2. This means all the two electrons are contained in an s orbital
Lithium 3Li = 3 electrons. Electron configuration = 1s2 2s1 . This means the first s orbital in shell number 1 is full so we have to put the next electron in shell number 2 s orbital.
Carbon =6C = 6 electrons. Electron configuration (EC) = 1s2 2s2 2p2 . The s orbital in the second shell 2 is full so the excess was put in a p-level 2p level. However, this there are three orbitals or subshells in this level [px, py, pz]. Therefore, we can write properly as 1s2 2s2 2px1 2py1 . . Furthermore, multiplet orbitals such as p orbitals must be filled with single electrons first before pairing them [HUNDS rule] = orbitals of the same energy (degenerate) must be filled singly before pairing.
Nitrogen =7N = 7 electrons. EC= 1s2 2s2 2p3 or 1s2 2s2 2 px1 2py1 2pz1 .
Oxygen = 8O = 8 electrons. EC = 1s2 2s2 2p4 or 1s2 2s2 2 px2 2py1 2pz1 .
There is a simple way to learn how to write electron configuration;
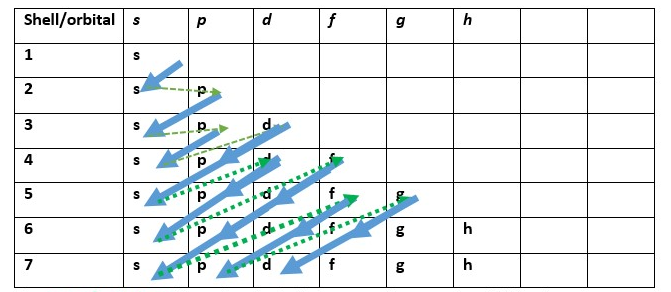
The green line LINKS the ending to the beginning of a new orbital filling [e.g . from 1s we go to 2s then the green line connects to 2p . We start at 3s green line connect to 3p then we go to 4s orbital. The next filling starts at 3d then to 4p then ends at 5s. This represents the beginning of diffusing or overlap of orbitals of different levels at the shell number increases].
Titanium= 22Ti = 22 electrons. EC= 1s2 2s2 2p6 3s2 3p6 4s2 3d2 . There are 5 orbitals, so the simple way is to use a box.
EC =1s2 2s2 2p6 3s2 3p6 4s2 3d2 . We can show how we fill this by using boxes to represent some of the the orbitals.

Sometimes a shorthand form of electron configuration is written by using the noble gas element that comes before the element is found e.g. Argon is the noble gas that comes before Titanium so we can shorten the electron configuration of Titanium as [Ar core =18 electrons 1s2 2s2 2p6 3s23p6 ] + valence EC. Therefore, the shorthand abbreviation for writing is;
EC of Titanium = [Ar] 4s2 3d2
The outer shell configuration is shown in boxes that starts at 4s.
Nickel = 28Ni = 28 electrons. EC = 1s2 2s2 2p6 3s2 3p6 4s2 3d8

Only the outer shell configuration is shown in boxes in this example for nickel.
Shorthand EC = Nickel = 28Ni = [Ar] 4s2 3d8
SPECIAL CASE: EXACTLY HALF FILLED AND FULLY FILLED MULTIPLET ORBITALS:
Multiplet is referring to degenerate orbitals such as p, d, f etc. This excludes the s-orbital.
Half-filled or fully filled orbitals are more stable and thus preferred e.g .
Chromium = 24 Cr = 24 electrons. WRONG EC = 1s2 2s2 2p6 3s2 3p6 4s2 3d4
[This is a special case, because a half-filled d-orbitals 3d5 gains extra stability and is preferred than 3d4 therefore we have to remove an electron from the 4s orbital and put it into the 3d orbital to make it half-filled 3d5 stable system, then any extra electron goes into the 4s before we start filling the 3d orbital again to pair electron] .
CORRECTED EC = 1s2 2s2 2p6 3s2 3p6 4s1 3d5

Valence shell contains 4s1 3d5
Short-hand EC = 24 Cr =[Ar] 4s2 3d 5
The half-filled d-orbitals in 3d5 give stability than 3d4
Copper = 29Cu = 29 electrons. WRONG EC = 1s2 2s2 2p6 3s2 3p6 4s2 3d9
[This is a special case, fully filled d-orbitals will give extra stability, it is preferable to remove a 4s electron and add it to the 3d orbital to get a fully filled 3d10, then any extra available electron goes into the 4s in the next element on the periodic table; that is Zinc =30Zn]
CORRECTED EC = 1s2 2s2 2p6 3s2 3p6 4s1 3d10

Valence shell contains 4s1 3d10
Short-hand EC = 29 Cr =[Ar] 4s1 3d10
Fully filled d-orbitals in 3d10 is more stable than 3d9
HOW ELECTRON CONFIGURATION AFFECTS PERIODIC TABLE OF ELEMENTS:
The highest shell number in the electron configuration of an atom represents the periodic position on the periodic table e.g. Nickel has shell number 4 as the highest shell number so nickel is found on period row 4 on the periodic table while Nitrogen and Oxygen are found on period row 2 because their highest shell number is 2 in the electronic configuration.
The type of orbital containing the valence or outer electrons determines the block position of the element on the periodic table [e.g. Lithium is s-block because its valence electrons are located in s-orbital while Carbon is in p-block because its valence electrons are located in p-orbitals. Nickel and Titanium are located in d-block since they have valence electrons in d-orbitals.
The group or column number is being determined from the number of valence electron in the valence orbitals. [e.g. Lithium is found in main group 1 column because it has only one valence electron located in s-orbital. Carbon is in group or column 4 because it has four valence electrons all located in p-orbitals.
However, the d-block elements are separated called TRANSITION ELEMENTS or METALS and are grouped separately e.g. Scandium, Sc has one valence electron in d-orbital so all the elements in the group or column containing Scandium have only one d valence electron. Nickel, Ni, is located on transition element group 8 because of 8 electrons in d valence orbitals.
Lanthanides and Actinides have f valence orbitals e.g. Cerium
Cerium = 58Ce = 58 electrons. EC = 1s2 2s2 2p6 3s2 3p6 4s2 3d10 4p6 5s2 4d10 5p6 6s2 4f2
The valence shell EC starts at 6s orbital, and the two valence electrons shows that Ce appears second in the row in the lanthanide series.
Thorium = 90Th = 90 electrons
EC = 1s2 2s2 2p6 3s2 3p6 4s2 3d10 4p6 5s2 4d10 5p6 6s2 4f14 5d10 6p6 7s2 5f2
The exact identity of an element is determined by the number of protons in the atom of an element; however, we can also use the number of electrons in the neutral atom of the element and the electron configuration (EC) to predict the chemical properties and position on the periodic table. This is because the elements on the table are arranged according to the atomic number or proton numbers and the chemical properties which also depends on the valence electrons in the atom.
ELECTRON TRANSITIONS IN THE ATOM AND ELECTROMAGNETIC SPECTRUM
Electromagnetic energy of attraction exists between a positive central nucleus and negatively charge ELECTRON in shells.
Energy (E) of attraction between nucleus and electron is related to 1/r2 where r is distance of the electron from the nucleus. In the same way, the quantized Energy (En) of electron in shell is related to 1/n2 where n is the shell number of the electron in the atom.
A higher Energy of attraction means the electron is tightly held by effective nuclear attraction. The electron shell is very close to the nucleus. Therefore, highest quanta of emission energy ΔE means smallest value of n (shell number 1) in any atom. This means in the emission of photons of energy ΔE, the electron at energy level En emits the highest energy photon if it falls to a final level n=1 lowest energy level.
En = -hcR/n2 where hcR are constant.
where h =Plank’s constant, c = speed of light, and R=Rydberg constant =109677cm-1

Electrons in lowest energy level have strongest attraction of energy [large negative or low positive En values] while electrons in high energy levels have low energy of attraction to nucleus [ low negative or high positive En values].
Electrons absorb energy to move to higher energy level but emit energy to move to lower level.
Energy emitted or absorbed = gap between energy levels = ΔE =hν
Energy is emitted in the form of photon with energy equal to ΔE = hν
Frequency = ν = c/λ , ΔE=hc/λ , 1/λ= ύ
ΔE = – [E2-E1] negative sign for emitted energy
En = -hcR [1/n2]
ΔE = – hcR [1/n22-1/n12]
ELECTROMAGNETIC SPECTRUM
The energy of emitted photons is related to distance from the nucleus and the size of the nuclear attraction. Therefore, GAMMA RAYS; photons emitted from within the nucleus in a nuclear decay process, have lots of energy and also X-RAYS; high energy photons emitted from powerful electronic transitions from tightly bound inner core shells closer to the nucleus in large metallic elements. On the other hand, visible light radio waves and microwaves have lower energies.
Number of photons in emitted radiation of energy E is given by N = E/hν
IONIZATION ENERGY (I) = ΔEI = E1-E2
Ionization energy is energy required to completely remove an electron from the atom.
Calculate the ionization energy of the hydrogen atom for n=1 and an atom for n=2
CHEMICAL BONDING
Atoms associate by sharing or transfer of electrons. Bonding occurs because atoms need to be isoelectronic with noble gas elements to achieve stability.
IONIC BONDING
Ionic bond usually occurs between metal and non-metal atoms. The loosely held valence electrons in metal atom is lost so the metal atom gets a net positive charge to become a Cation but the non-metal atom gain electrons and acquires a net negative charge to become an Anion. The two species (negative and positive ions) form a bond by a strong electrostatic force of attraction between them.
The example illustrates formation of sodium cation (Na+) and Chloride Anion (Cl–) from their parent atoms and ionic bond formation in Sodium Chloride NaCl.
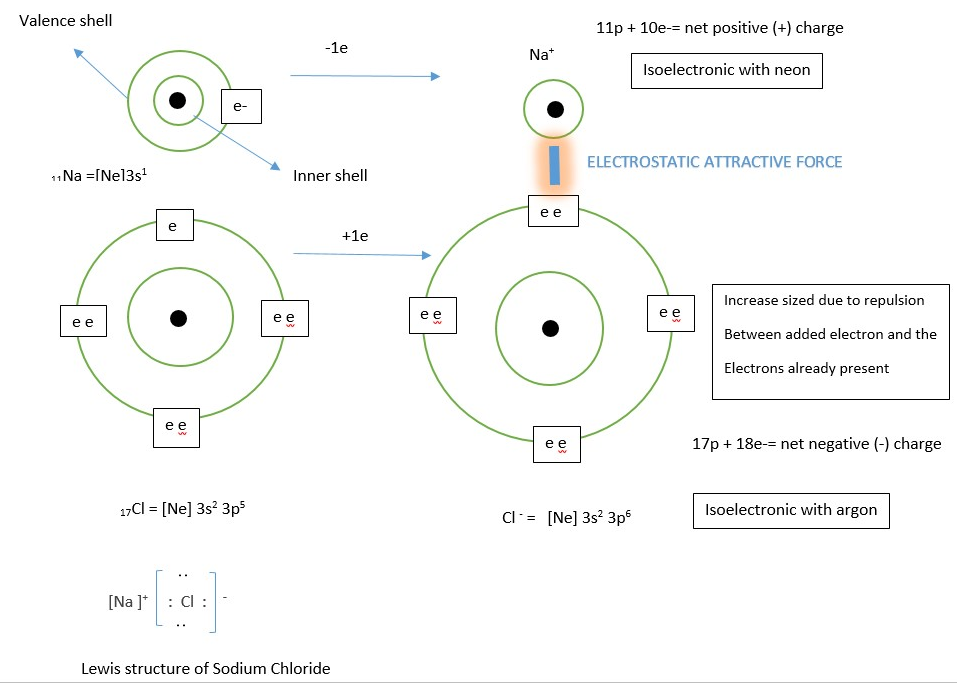
Cations are usually smaller than their parent atom due to increased effective nuclear charge, but anions are usually larger the parent atom due to increased repulsion between the electrons in valence shell when a new electron is added.
The other example is the formation of ionic bond in Magnesium oxide MgO
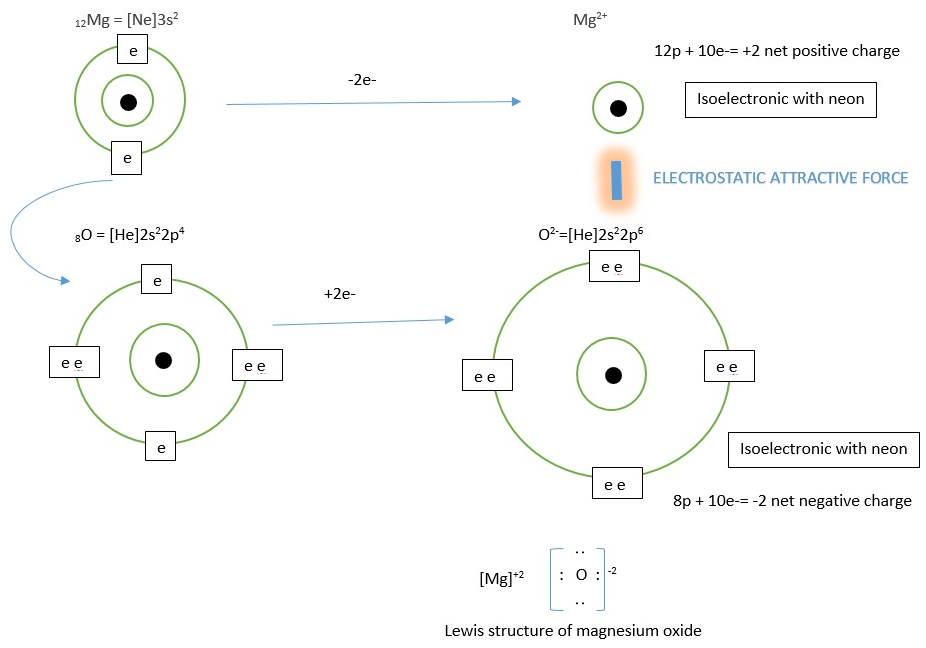
Try illustrations with MgCl2 and Na2O
Covalent bonding
This occurs when two atoms associate by sharing of valence electrons. The shared electron cloud becomes part of the electron cloud around the atomic nuclei that holds the molecule together. In order for covalent bonding to occur, the atoms must approach each other at a bonding distance. This type of bonding usually occurs between two non-metal atoms. The shared or bonding electron pairs are usually represented by a DASH line in Lewis’s structure diagrams. A typical example is chemical bond from two hydrogen atoms by sharing electrons to form H2 gas molecule.
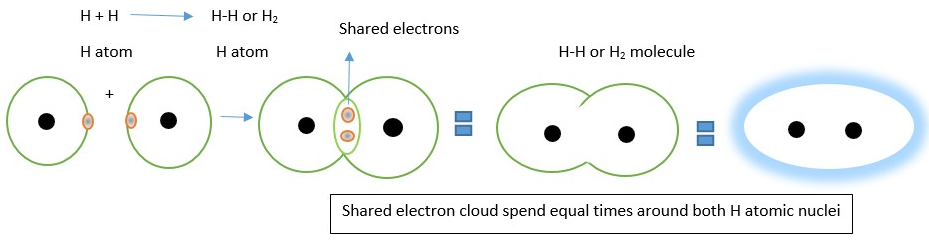
Another example is formation of F2 molecule by covalent bond from two Fluorine atoms.
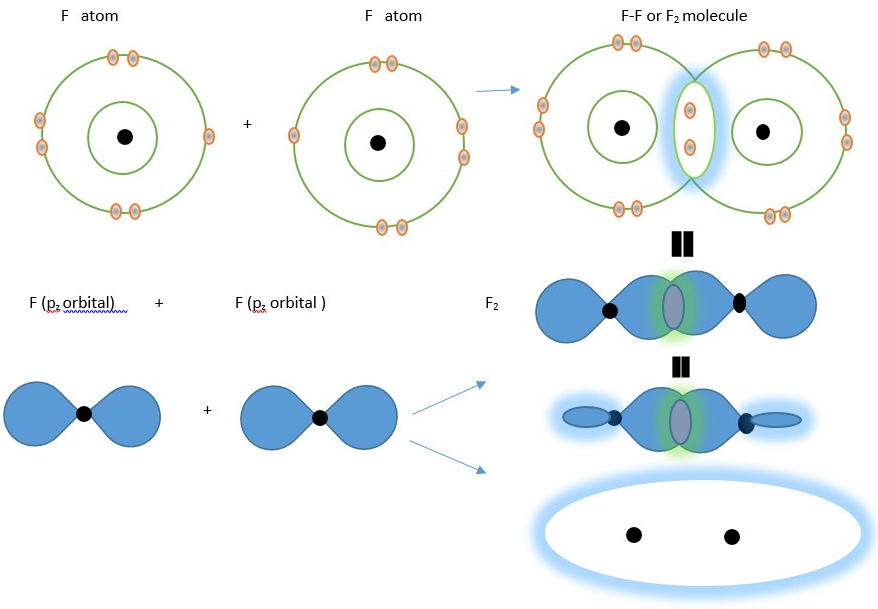
The arrows and equal signs refer to different representation of the same F2 molecule. The first dot and circle diagram of the atoms and molecule is the old classical way of showing atoms and the covalent bond between atoms. The 8 electrons around each F nucleus in F2 makes each F atom isoelectronic with noble gas neon in the F2 molecule.
The electron orbital diagram gives a better illustration;
F= [He]2s22p5 or [He]2s2 2px2 2py2 2pz1
Each Fluorine atom shares the single unpaired electron located in a p-orbital to form F2 molecule. The p orbitals must be in correct orientation and distance to overlap to form a covalent bond. Therefore, a pz orbital must overlap with pz orbital py orbital must overlap with py and, px orbital with px.orbital for good overlap and bond formation due to having same orientation in space.
Try illustrations with formation of Cl2 and Br2
In formation of hydrogen fluoride HF below, the illustration is showing the first simple classical view using valence shells as circles and dots as electrons of H and F as used in Lewis’s structure diagrams. The second is showing covalent bonding using atomic orbital diagrams [i.e. s-orbital of H and one p-orbital of F].
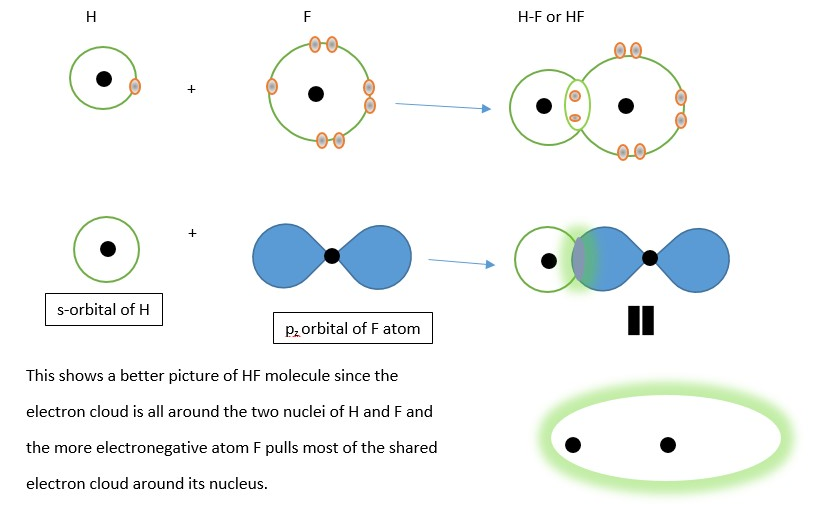
SPECIAL CASE:
Covalent bonding involving three or more atoms.
-
- Covalent bonding and the structure of the Water molecule H2O ;
2H atoms + 1 O atom
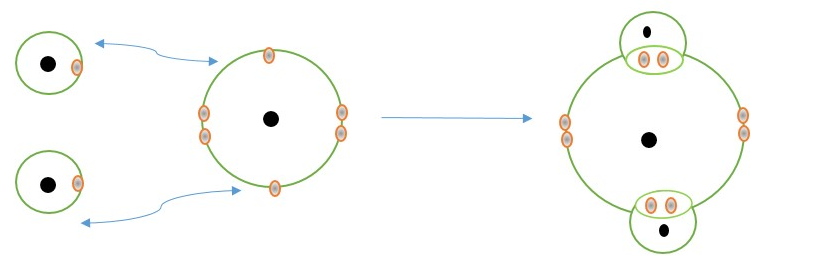
WRONG : This shows water molecule can be linear (H-O-H) when formed.
Orbital diagrams
1H =1s1 + 8O =[ He] 2s2 2p4
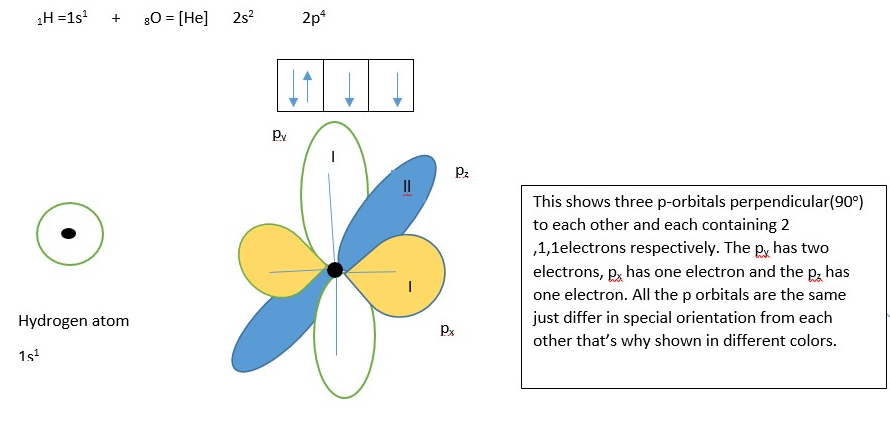
The orbitals containing single unpaired electrons will be used for bonding with two hydrogen atoms. The assumption is that, py and pz forms the covalent bond while pz is not used.
WRONG : The water molecule forms H-O-H bond angle that is right angled perpendicular.
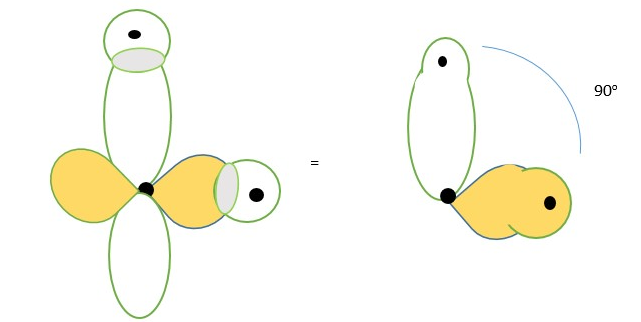
This shows and predicts water H-O-H bond angle to be 90 degrees which is not what is observed from experiments. Experiments shows a water molecule is V shaped with a larger H-O-H bond angle close to 109o. This is also the case for several other known compounds (such as METHANE CH4 and AMMONIA NH3 etc ) when we use the raw atomic orbitals. This shows that in the case of covalent bonding involving three or more atom or formation of double or triple bonds between two atoms, we cannot just use the raw atomic orbitals to predicts the shape and bond angles of the molecule accurately.
CONCEPT OF HYBRIDIZATION
This refers to the idea of mixing of atomic orbitals in the atom to form new molecular orbitals before forming a bond with another atom. The new molecular orbitals in the atom are called HYBRID molecular orbitals and they have different orientation in space from the original atomic orbitals from which they are made. Using the idea of hybridization in formation of water molecule gives the correct structure that is V shaped close to bond angle of 109-degree H-O-H bond angle. The valence orbitals in Oxygen atom mix first to form new hybrid molecular orbitals before forming bond with Hydrogen s-orbital electrons.
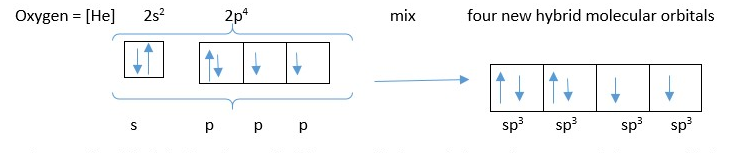
The atomic orbitals in the valence shell [ one s-orbital containing 2 electrons, and three p –orbitals containing 2,1, and 1 electron respectively] all mix up to form four new molecular orbitals (four sp3 hybrid molecular orbitals) equal in length and size and oriented in space at tetrahedral angle of 109 from each other. Two of the orbitals contain two electrons each while each of the other two contain a single electron each.
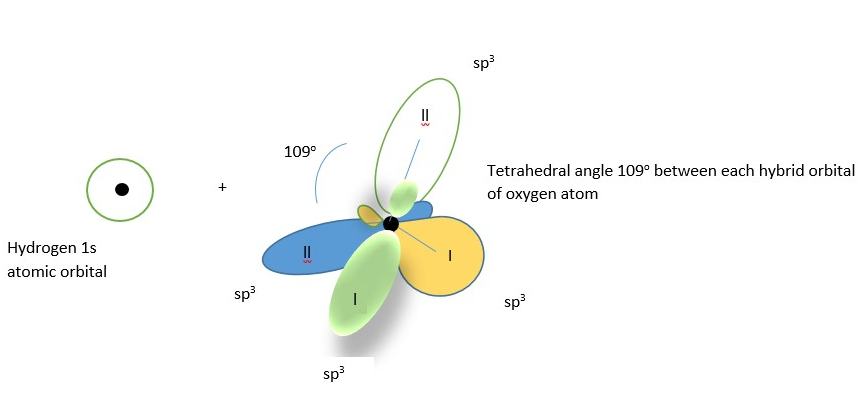
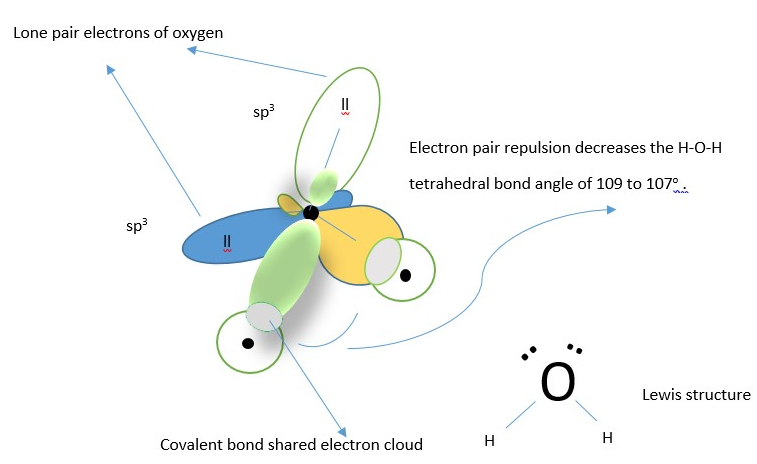
The hybridization of atomic orbital helps to justify the observed experimental bond angle in water molecules.
NOTE:
Ignore shell number in front of the letter (e.g., 1s and 2p) and also ignore the superscript numbers of electrons behind the letters (e.g. s2 or p4) but just count only the orbital alphabets used in the hybridization mixing. [e.g an s orbital mixes with a p-orbital to form two new sp hybrid molecular orbital; Just like 1+1 =2 or 1 + 2 =3]. Share the number of electrons in the original atomic orbitals for the new molecular orbitals. However sometimes excitation and promotion of electrons should occur before mixing the atomic orbitals.
The other example is formation of methane. Experiments show the H-C-H bonds are arranged tetrahedrally(109o) and not perpendicular (90o) from each other. Why? Hybridization
WRONG STRUCTURE:
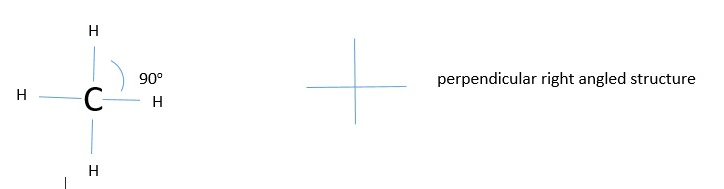
CORRECTED STRUCTURE:
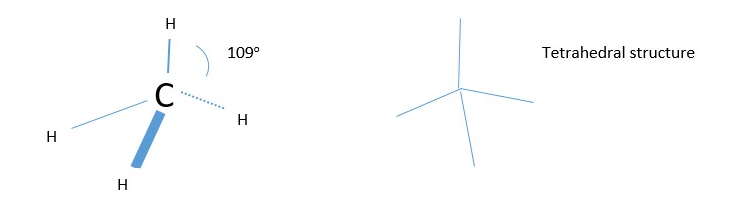
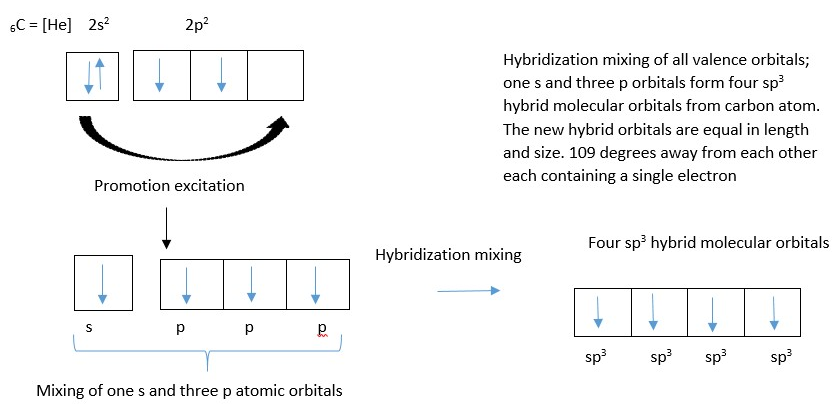
Excitation energy is needed to unpaired the lone pair and promote one electron into an empty p orbital before hybridization occur in this case.
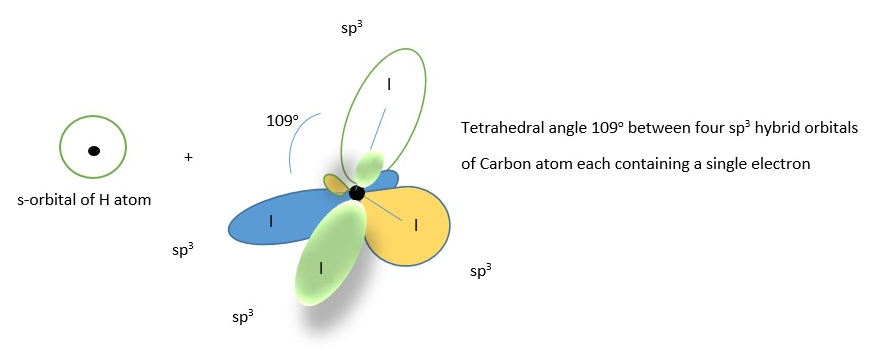
Four s orbital electrons of four hydrogen atoms form covalent bonds with four sp3 hybrid orbitals of Carbon atom to form the CH4 molecule shown below .The bonds of the same in length and size and all the H-C-H bond angles are the same 109 through out.
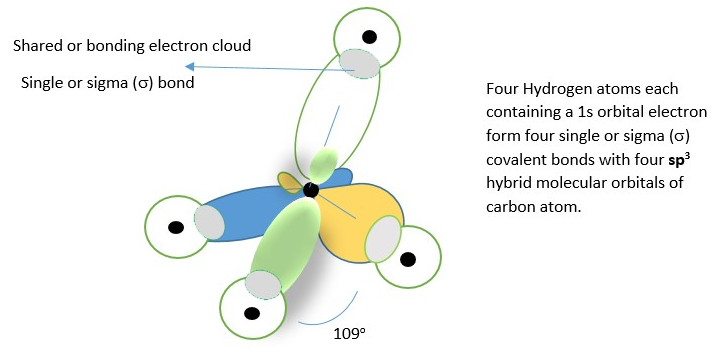
If there was no hybridization, the s-orbital of carbon atom will form a bond with s-orbital of hydrogen atom. The other three p-orbitals will form bonds with s-orbital of three hydrogen atoms. This means, one of the bonds (s-s covalent bond) will be different length and size and spatial orientation from the other three (s-p covalent) bonds if we don’t use the concept of hybridization to predict the structure. However, experiments shows that all the bonds are the same in length, size and orientation in space, so this proves hybridization is helpful in predicting the structure of organic molecules.
Other examples include CO2, H2CCH2, H2CO , CO
Experiments show CO and CO2 are linear molecules, but ethane and formaldehyde have HCC and HCO bond angles of 120 respectively. This is explained by using idea of hybridization.
Covalent bonding in CO2 formation:
Hybridization of the carbon in CO2 ;
The Carbon forms an sp hybrid molecular orbital.
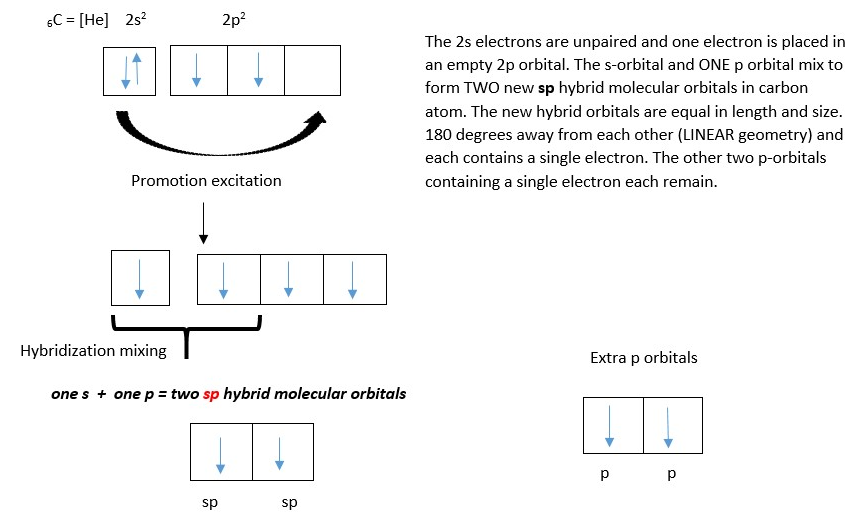
Carbon atom showing two LINEAR sp hybrid molecular orbitals (in blue) and two unhybridized p-orbitals (no color).
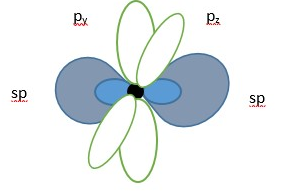
Two sp hybrid molecular orbitals in carbon atom.
Hybridization of the atomic orbitals in the oxygen atom is slightly different and several possibilities are possible for the two oxygens to form doubles bonds with the carbon atom.
First is the use of raw unhybridized p-orbitals in the oxygen atom.
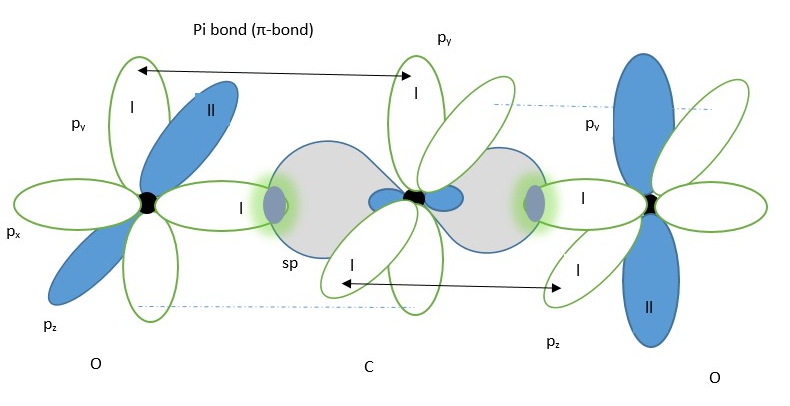
The second is oxygen atom forms an sp hybrid molecular orbital before bonding with the carbon atom.
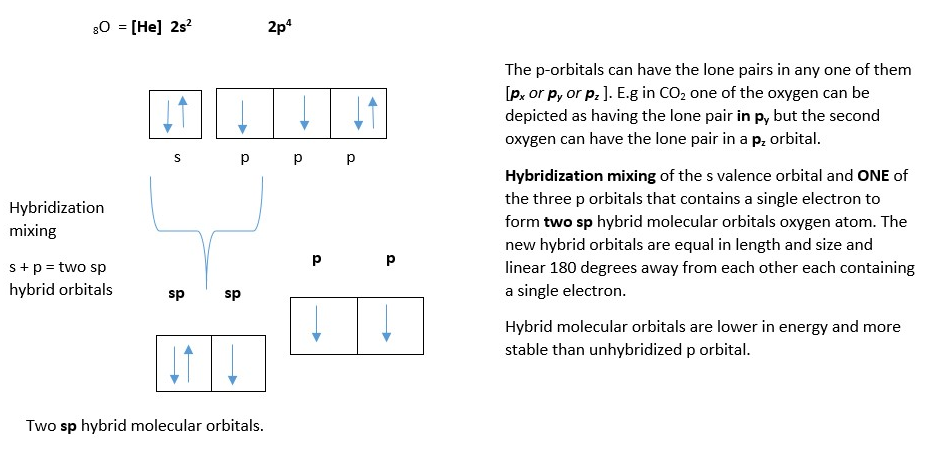
Oxygen atom showing two LINEAR sp hybrid molecular orbitals (in yellow) and two p-orbitals.
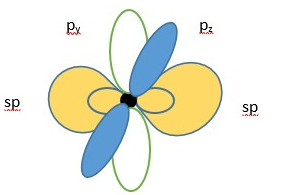
sp hybrid molecular orbital from carbon and oxygen each containing a single electron will overlap to form a covalent sigma bond (shown in the middle). The p orbitals containing a single electron from oxygen atom will overlap with that from carbon from the left side while the second oxygen p-orbital having a single electron bond overlaps with the second p-orbital on the carbon from the right side to form covalent pi-bonds above and below the nuclei.
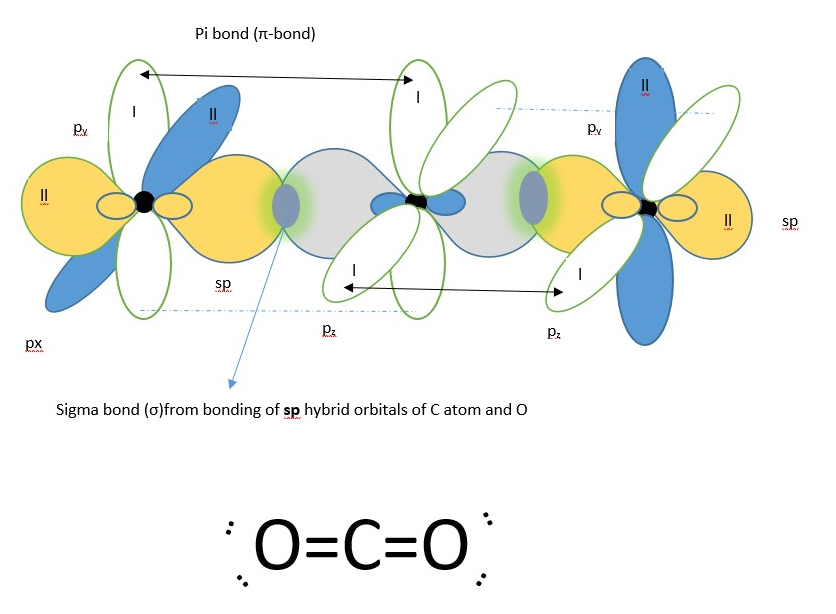
Lewis structure
The last possibility is oxygen atom forms an sp2 hybrid molecular orbital before bonding with the carbon atom.The other possibility is for the oxygen to form an sp2 hybrid molecular orbital instead of sp orbital. In this case there is only one un-hybridized p-orbital left on the two oxygen atoms (py or pz).
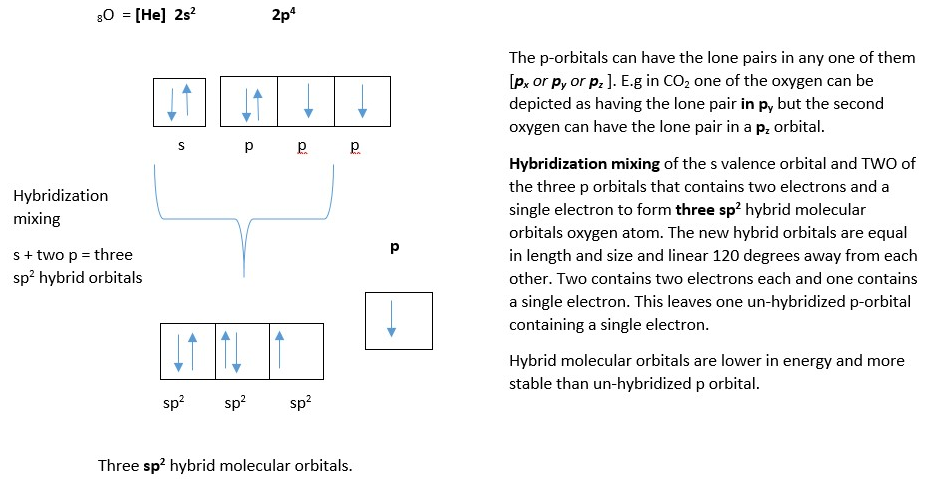
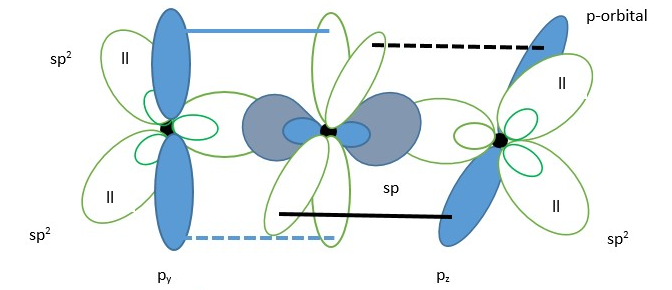
Based on the rule for stability of electron orbitals, molecular orbitals and bonding orbitals = [s>sp>sp2>sp3………>p] …. decreasing stability. Thus, structure with high s/p ratio percentage character are more stable and preferred in nature. This because s-orbitals are closer to the atomic nucleus and thus stabilize electrons more than other orbitals.
ENERGY LEVEL DIAGRAM FOR S AND P AND THEIR HYBRID AND BONDING ORBITALS
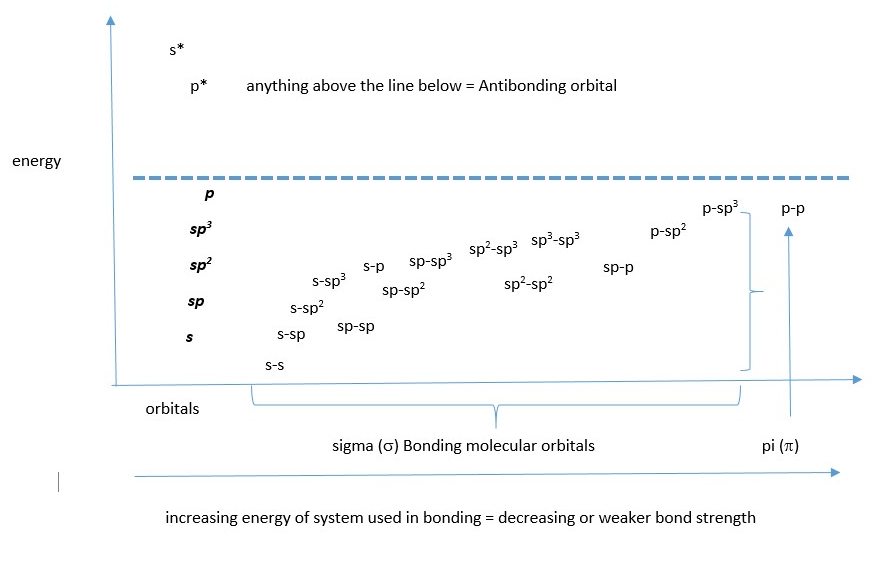
We can choose which system will give the lowest energy structure for CO2 molecule. All three bonding examples for CO2 listed above have the carbon atom with an sp hybrid molecular orbital so we focus on the oxygen atoms. In the first possibility example, the oxygen has lone pair in un-hybridized p-orbital which raises the energy and it uses p orbitals to form sigma bonds with sp hybrid orbital of carbon. This gives a very high p character for the bonds (weaker sigma bonding) in the compound compared to the other two possibilities. The second possibility has the sp hybrid orbital of oxygen forming a good overlap with the carbon sp hybrid orbital (high s character ;sp+sp =50%s), however it has lone pair in un-hybridized p orbital and that raises the energy of the system. The last example does not have this issue because the lone pairs are in stable sp2 hybrid orbitals, and the bonds have some s character (sp2+sp=40%s).
Based on these reasons, the best choice is the last possibility where the oxygen is sp2 hybridized because it gives the lowest energy structure for neutral CO2 molecule.
Covalent bonding in Carbon monoxide; CO formation
Both carbon and oxygen form an sp hybridized molecular orbital, but there is formation of triple bond because of only one Oxygen atom. CO is a linear molecule.Therefore, the hybridization in carbon atom changes slightly in that there is no promotion of s electron into a p-orbital but instead the orbital mixing just occurs;
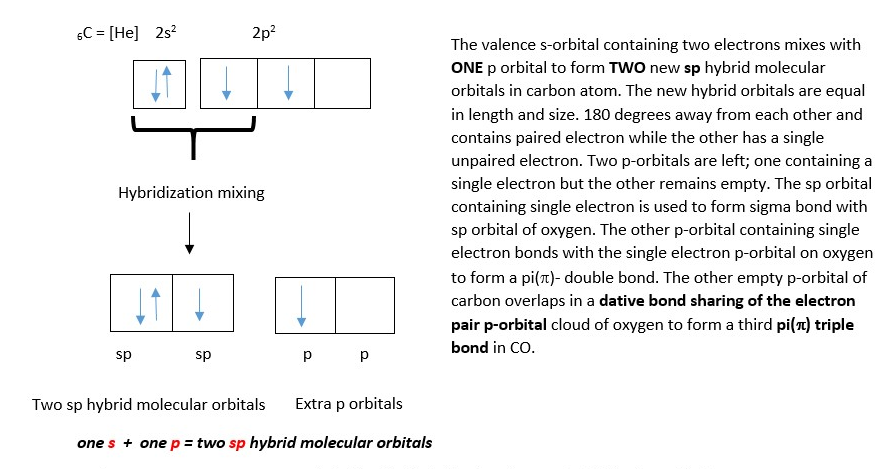
Carbon atom gets two LINEAR sp hybrid orbitals (in blue) and two unhybridized p-orbitals.
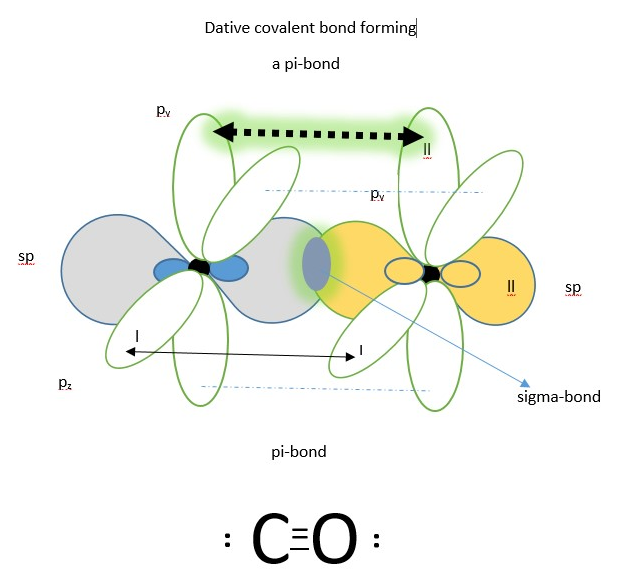
Lewis structure
NOTE: There is another plausible explanation in the literature suggesting that oxygen transfers one of the p-orbital electrons to carbon then both atoms form sp hybrid molecular orbitals before covalent bonding to form one sigma bond and two pi-bonds. This also makes lots of sense.
OTHER EXAMPLES :
The other example is formation of ethene (H2CCH2) and formaldehyde where the carbon forms hybridize its atomic orbitals to form an sp2 hybrid molecular orbitals before bonding with another atom.
The other example is formation of ethene (H2CCH2) and formaldehyde where the carbon forms hybridize its atomic orbitals to form an sp2 hybrid molecular orbitals before bonding with another atom.
Covalent bonding in formation of Ethene :
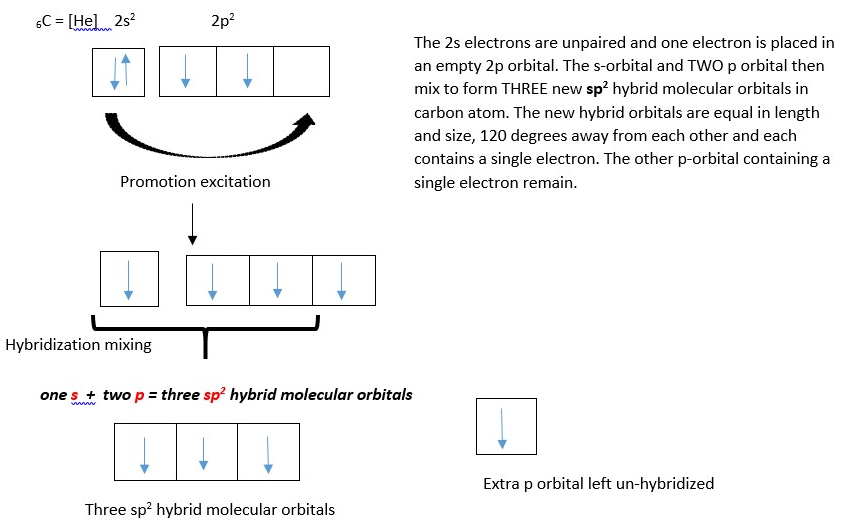
Left: Carbon atom showing THREE sp2 hybrid orbitals (TRIGONAL shape). Right: Three sp2 hybrid molecular orbitals and one unhybridized p-orbital (in blue).
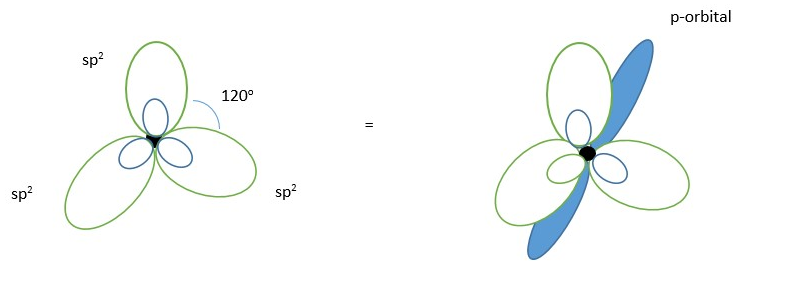
One sigma bond is formed by overlap of two sp2 orbitals from each carbon. The un-used p orbitals on each carbon form overlap to form a pi-bond while the remaining sp2 hybrid orbitals on each carbon overlap with the s orbitals of Hydrogen to form four sigma bonds.
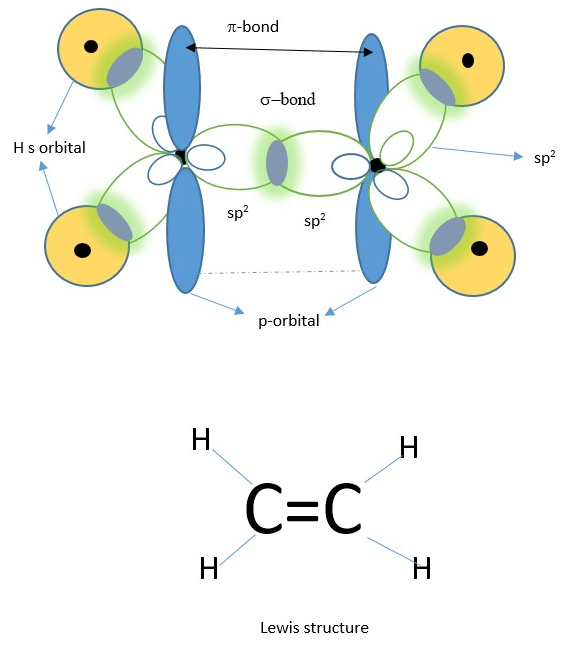
COVALENT BONDING IN ETHYNE FORMATION
Ethyne or acetylene is a linear molecule due to hybridization of the carbon atoms. The carbon hybridizes its atomic orbitals [one s-orbital and one p-orbital] to form two sp hybrid molecular orbitals before bonding with another atom.
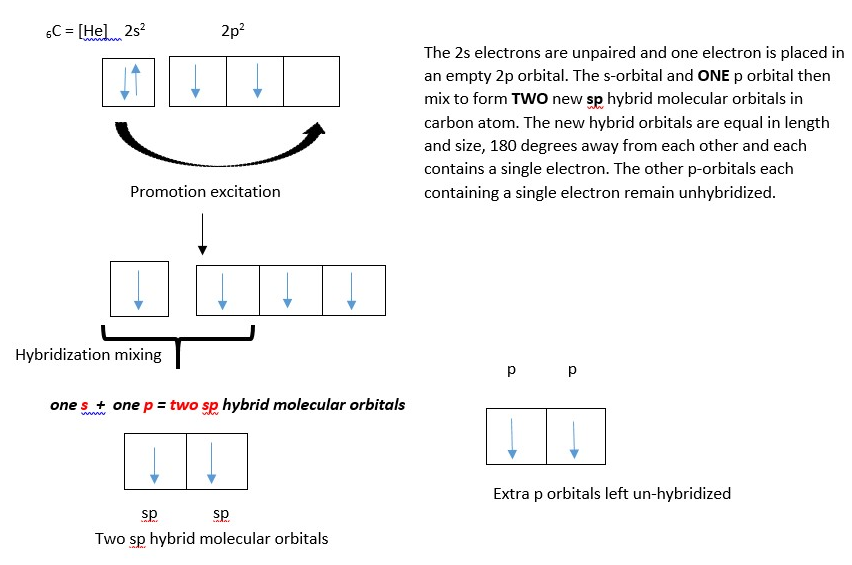
Carbon atom showing two LINEAR sp hybrid orbitals (in blue) and two unhybridized p-orbitals.
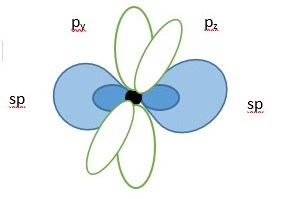
One sp orbital from each carbon form a sigma(s)- bond (center) but the other sp on each carbon form a sigma bond with s-orbital of hydrogen atoms. The unhybridized p-orbitals each containing a single electron overlap to form a pi-bond above and below the nuclei. This explains why ethyne a.k.a. ( acetylene) is a LINEAR molecule and has a triple bond [i.e. one sigma bond and two pi-bonds].
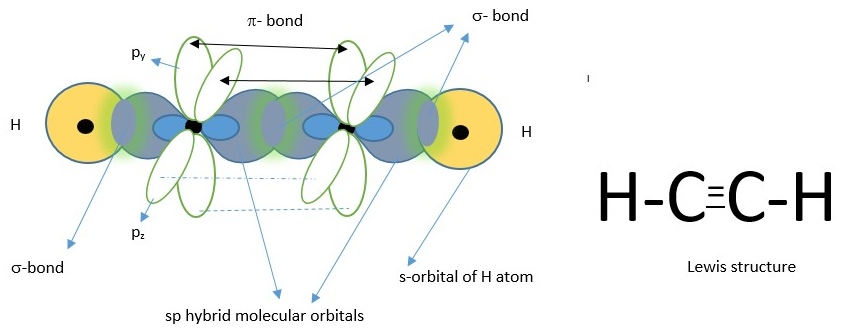
Dative or coordinate covalent bonding.
This is type of bonding in which the bonding electrons are given by only one of the atoms to an empty orbital of another atom. Examples of this occur in carbon monoxide (CO) and in ammonium NH4+ ion. CO is already discussed above however you can try and figure out coordinate bonding in NH4+ by completing sp3 hybridization of nitrogen and use the lone pair on nitrogen to bond with hydrogen ion. This is also the primary way by which non-metal molecules bond to metal atoms. E.g . metal carbonyl bond M-C=O